Fundamental Catalysis Enabling a Zero-Carbon-Footprint Future
By Johannes Lercher
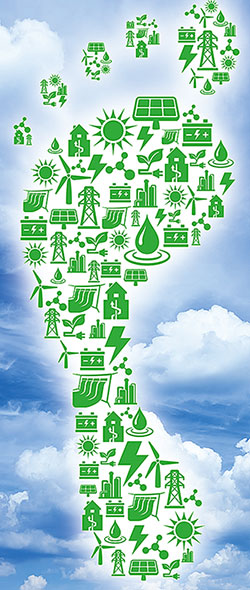
Economic wealth is directly correlated with the energy used to perform work, enable mobility, and provide the goods required to ensure the well being of individuals. Today, more than 80 percent of chemicals and energy carriers are synthesized from fossil (hydro)carbon resources, which has led to an unprecedented increase in the levels of carbon dioxide (CO2) in the atmosphere. Nearly all these chemicals and energy carriers are made using catalysis in at least one of the processing steps. Catalysis is, therefore, not only key to sustainable development, characterized by transition from the current fossil carbon-based energy carriers to a zero-carbon footprint energy future, but it will also secure energy independence.
On a global scale all energy demands can potentially be satisfied without using fossil carbon resources by harvesting solar energy directly or via wind, hydropower, and photosynthetically produced biomass. Tapping increasingly into these resources will lower the net CO2 emission, the so-called carbon footprint. The problem arises, however, from the fact that this energy can only be harvested in a highly distributed form as electricity, which is challenging to store and would require an unrealistically large investment in the infrastructure (e.g., to power vehicles), if used as the sole energy form.
The direct application of today's strategy to produce energy carriers and chemicals, to rely on the economy of scale will not be transferable into the future. Combining hydrogen generation via photo- or electrocatalysis (e.g., from wind or water power) with the reductive conversion of biomass to energy carriers and chemicals in conventional large-scale plants is uneconomical. It requires collecting the distributed energy and would force investing a large amount of energy to obtain the final product in return (energy return of energy invested, EROEI). Even in the best cases, between 30 and 50 percent of the energy produced has to be invested to make the energy carrier. Thus, catalysis faces the challenge to reinvent the large processes matured over decades to enable a viable synthesis of energy carriers at scales 100 to 1000 times smaller than today's chemical plants and refineries. This can only be done by developing new catalysts that can operate under less severe conditions, with higher rates and selectivities than previously possible. It would also require that the reduction equivalents in the form of electrons be linked in electrocatalytic processes to add hydrogen to renewable carbon sources and transform them into energy carriers and chemicals.
The approach
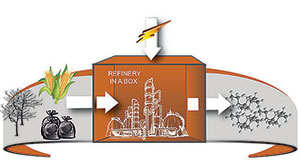
Distributed production of environmentally sustainable fuels and chemicals—chemical conversion at the source—can scale down refineries and processing plants enabling decentralized production close to energy sources and carbon feedstock. Operations under low temperatures and low-to medium pressure are safe and simple.
Several critical boundary conditions dictate the catalytic chemistries that have to be achieved to develop catalysts that can operate at lower temperatures and pressures and with higher selectivities. The lower temperatures require 1) operation primarily in condensed phase, 2) catalysts that enable the transformation of molecules with lower energy barriers and higher transition entropies, and 3) efficient transport and separation of molecules. Hydrogen from renewable energy is the critical reactant required to achieve the goal of a carbon-neutral synthesis of hydrocarbon energy carriers.
While the input for these boundary conditions certainly come from applied problems, new catalysts can hardly be developed through empirical trial-and-error approaches—the space to be explored is simply too large, even when applying the most advanced parallelization technologies. Central to the strategy of the Institute for Integrated Catalysis is, therefore, development of a principal understanding of the catalytic chemistries in three distinctive areas, which lay the basis for breakthroughs toward a zero-carbon-footprint future. These areas are:
- Storing electric energy in hydrogen (H2 production takes up +40 percent of direct energy requirements in many reductive petrochemical processes, a chemistry that is a focus of the Center for Molecular Electrocatalysis, an Energy Frontier Research Center at PNNL)
- Adding hydrogen to oxo-functionalized carbon resources (i.e., biomass components or CO2, which store in essence the solar energy in chemical bonds and allow use of existing chemistry and infrastructure)
- Cleaving and manipulating carbon-carbon and carbon-heteroatom bonds (i.e., ensuring the cleanest energy carriers possible and optimally produced chemicals).
The successes and the path forward
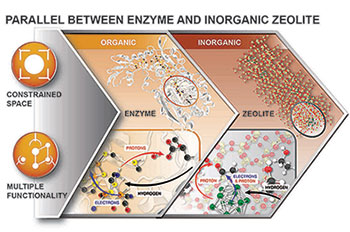
Institute of Integrated Catalysis scientists are transferring concepts used by enzymes to inorganic catalysts by designing active sites with multiple functionalities and tailoring chemical properties around them.
Key to the progress has been the linkages between the disciplines in catalysis, bridging surface science, heterogeneous, homogeneous, and increasingly, biocatalysis. Learning to speak a common language is beginning to help question paradigms and open creative ways to develop new catalytic approaches. A transdisciplinary approach has also been helpful in the rigorous studies of mechanisms of elementary transformations of key reactions, in probing experimentally and theoretically the elementary steps of catalyzed conversions at surfaces and at metal-organic complexes, and in understanding the breaking and making of bonds in molecules at the atomic level. Such studies have included, for example, enhanced rates of electrocatalytic reductions of protons to H2 with molecular catalysts (i.e., in the presence of a metal center with ligand groups enhancing H+ delivery), which is mirrored in the enhancement of hydrogenation rates by the simultaneous presence of metal and Brønsted acid sites, or the mechanistic understanding of site-specific reaction sequences during water elimination from ethylene glycol on single crystals and polycrystalline oxides.
In our aim to find the best routes to reduce oxygen-containing molecules to hydrocarbon fuels and CO2 to oxygenates, we analyze pathways for which the various reaction intermediates have a minimum variation in the free energies, hypothesizing that such a pathway has minimum barriers. We have identified two principles helping to bring this free energy of the ground states along pathways and to further enhance catalytic reactivity: multifunctional catalysis in which more than one site interacts simultaneously with the reacting molecules, and spatial as well as chemical tailoring of the space around the active site, which minimizes the free energy of the transition states.
Both are features of catalytic sites found in enzymes—features from which we wish to learn more intensely in the future, translating the principles of enzymes to robust inorganic catalysts. Inspiration can be taken for example from the ubiquitous multifunctionality of the active sites of enzymes, such as the heterobimetallic system in [NiFe] CO dehydrogenase or the incorporation of a pendant Lewis base in [FeFe] hydrogenase. Both examples also highlight the role of the accessible space and its functional properties, the adjacent functional groups, and the hydrophilic or lipophilic surfaces.
Overall, fundamental catalysis is set on a path to develop new transformative catalytic approaches, through understanding elementary principles, translating principles across disciplinary boundaries, and integrating these findings into the practical needs that have been sketched above. It is a path that will enable us to discover new principles and solve long-existing problems and challenge each of us, but also a path that allows us to make important contributions to the developments required by the inevitable changes of the energy future ahead of us.
Dr. Johannes Lercher is director of the Institute for Interfacial Catalysis at Pacific Northwest National Laboratory, and professor of chemistry at Technische Universität München, Germany.