
One Man's 'Process Intensification'
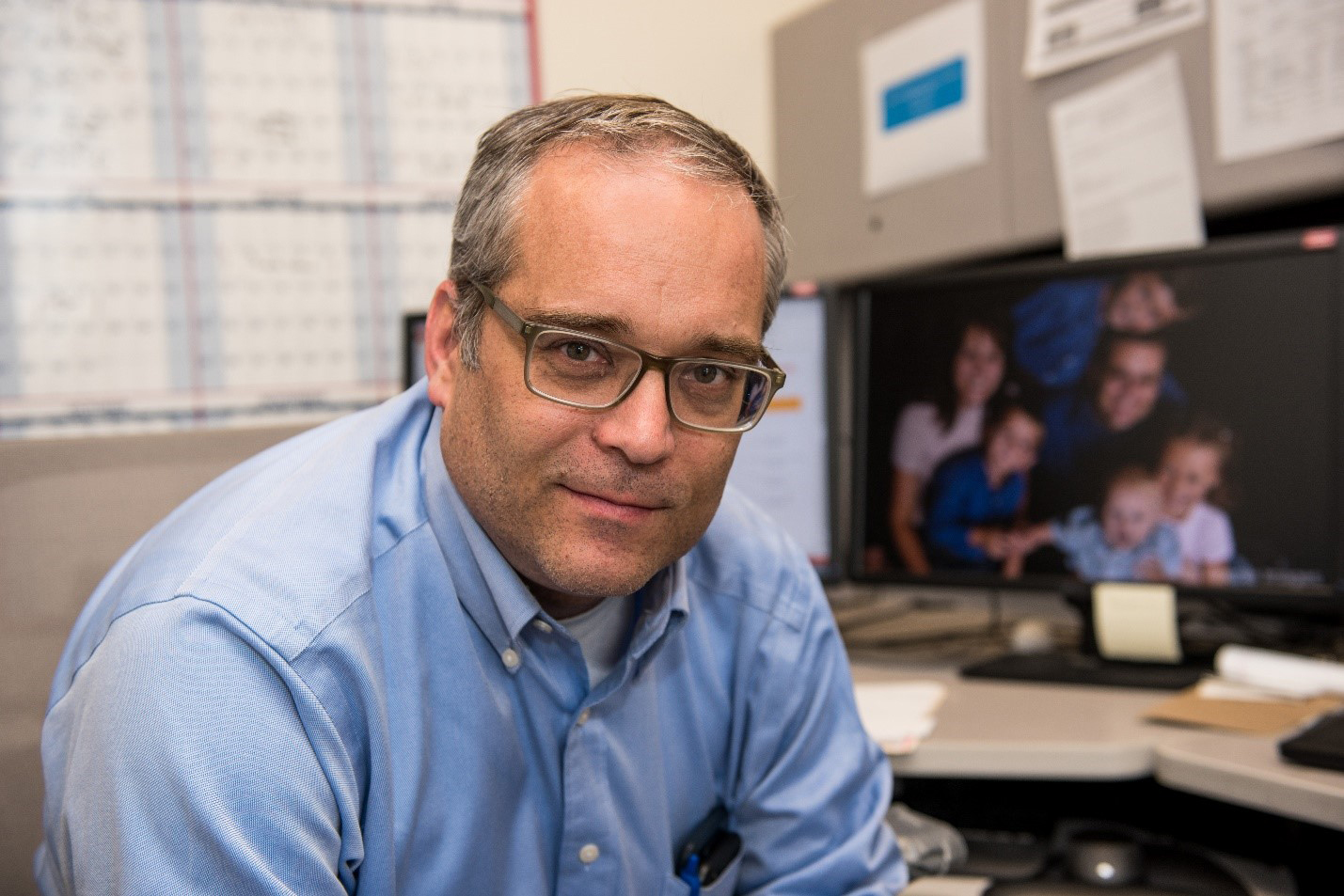
Jamie Holladay in his office at Pacific Northwest National Laboratory.
A renewable energy researcher pursues the fast, efficient, and inexpensive
Late one spring afternoon, chemical engineer Jamelyn "Jamie" D. Holladay stood up from his desk at Pacific Northwest National Laboratory (PNNL), swept on a jacket, and speed-walked down a corridor to the outdoors.
He was rushing to watch his son (one of six children) run the 800 meters and the 1600 meters at a high school track-and-field meet.
On his desk that day he left behind fresh copies of The Elements of Style, a classic American writing guide now a century old. Holladay hands them out to staff. "We become engineers, so we don’t have to write," he said. "Now (writing) is a big part of what we do. And on many days, it’s all I do."
Later that day, at the track, his son broke two personal bests. "He's a great distance runner," said Holladay. He could have been describing a version of himself: a researcher who for close to two decades has gone the distance–in his case, on behalf of a clean-energy future.
A chemistry buff even before his teens, the Philadelphia native studied lithium batteries with mentor John Harb at Brigham Young University in Utah (B.S. 1996, M.S. 2000). "I thought they were cool," he said of the batteries and went on to write a master's thesis on the lithium-ion variety.
Faster, Cheaper, Better
Holladay arrived at PNNL in 2000 and later earned a Ph.D. in chemical engineering (Washington State University, 2015). His dissertation advisor was Laboratory Fellow Yong Wang, who has a joint PNNL-university appointment and is a research collaborator of Holladay's.
Wang is well known for his catalysis and reaction engineering work. It's related to transforming fossil and biomass feedstocks into fuels and chemicals, as well as reducing vehicle emissions.
Holladay's own research path at PNNL has been nontraditional, "very broad, spanning many areas," he says. His curriculum vitae reflects work in hydrogen production, energy storage, emissions reduction, catalyst development, and the electrochemical systems underlying affordable clean vehicles.
Still, there is a unifying theme—"process intensification," he calls it—which has bent the arc of Holladay's career towards clean-energy technologies that are less expensive, more efficient, and faster.
"Engineers are the laziest people in the world," said Holladay. "We all look for ways to do things with less energy and less money."
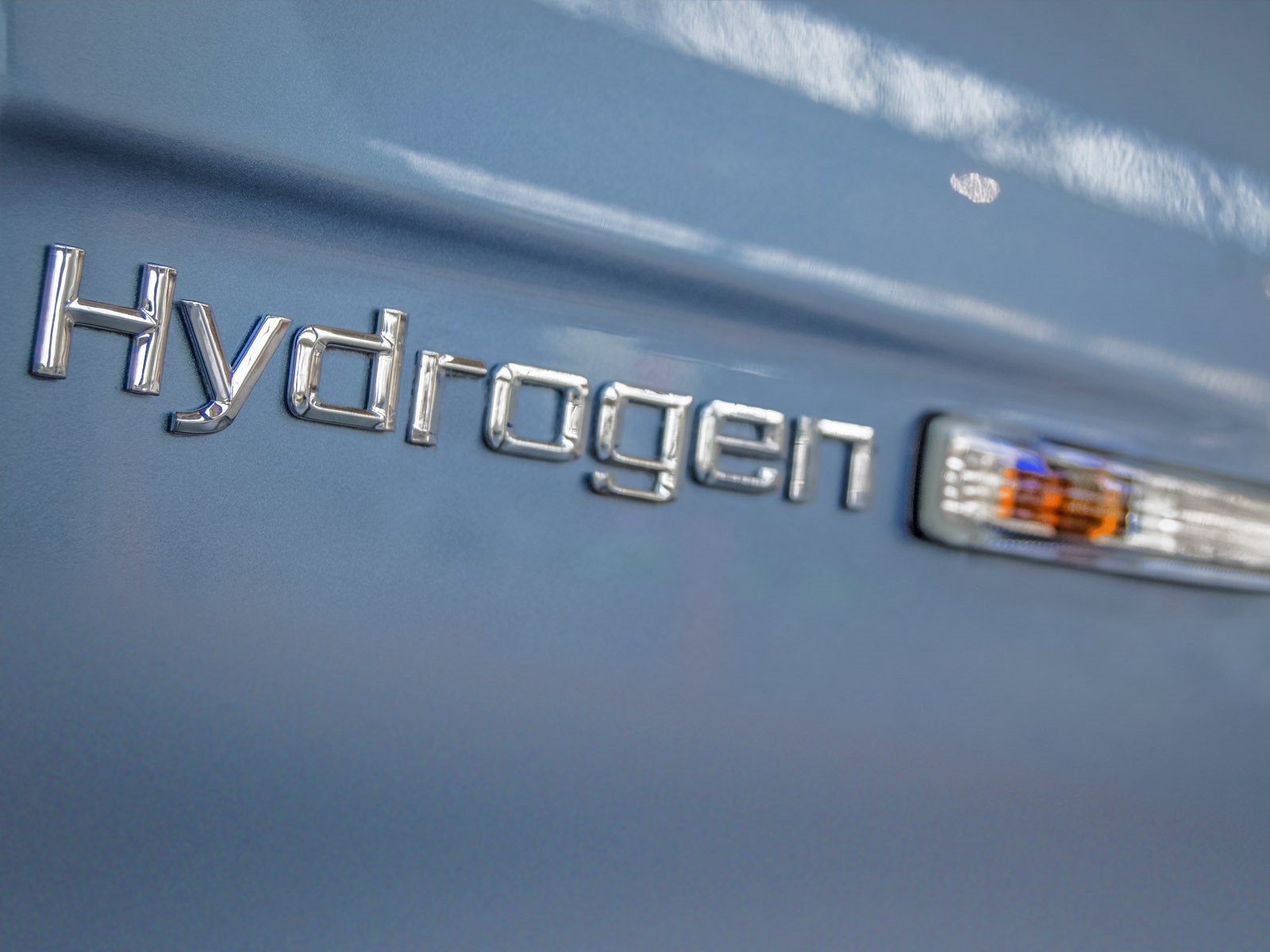
Holladay is interested in zero-emission vehicles, and says that almost all of the major car manufacturers are developing or selling hydrogen fuel cars.
The Work of Two Decades
Process intensification and catalysts run like a thread through Holladay's work at PNNL, where he is currently team lead for the Catalysis Science and Applications group.
He recently helped shape a project involving his PNNL colleague Robert A. Dagle, who is exploring better ways to get hydrogen out of the methane in natural gas. The process creates very little carbon dioxide, a greenhouse gas. "Most of the carbon goes to structural carbon—carbon nanotubes—that you can resell," he said of another benefit of the project. "You have two value streams."
The predominant shape of Holladay's PNNL identity comes from pursuing new technologies for renewable energy. Meanwhile, he is one of just a few representatives at PNNL for a government-industry partnership called U.S. DRIVE (Driving Research and Innovation for Vehicle efficiency and Energy sustainability).
Holladay is also the lab's sub-sector manager for the Department of Energy's Fuel Cell Technologies Office. "I help a lot of people get projects in place," he said.
Making Hydrogen
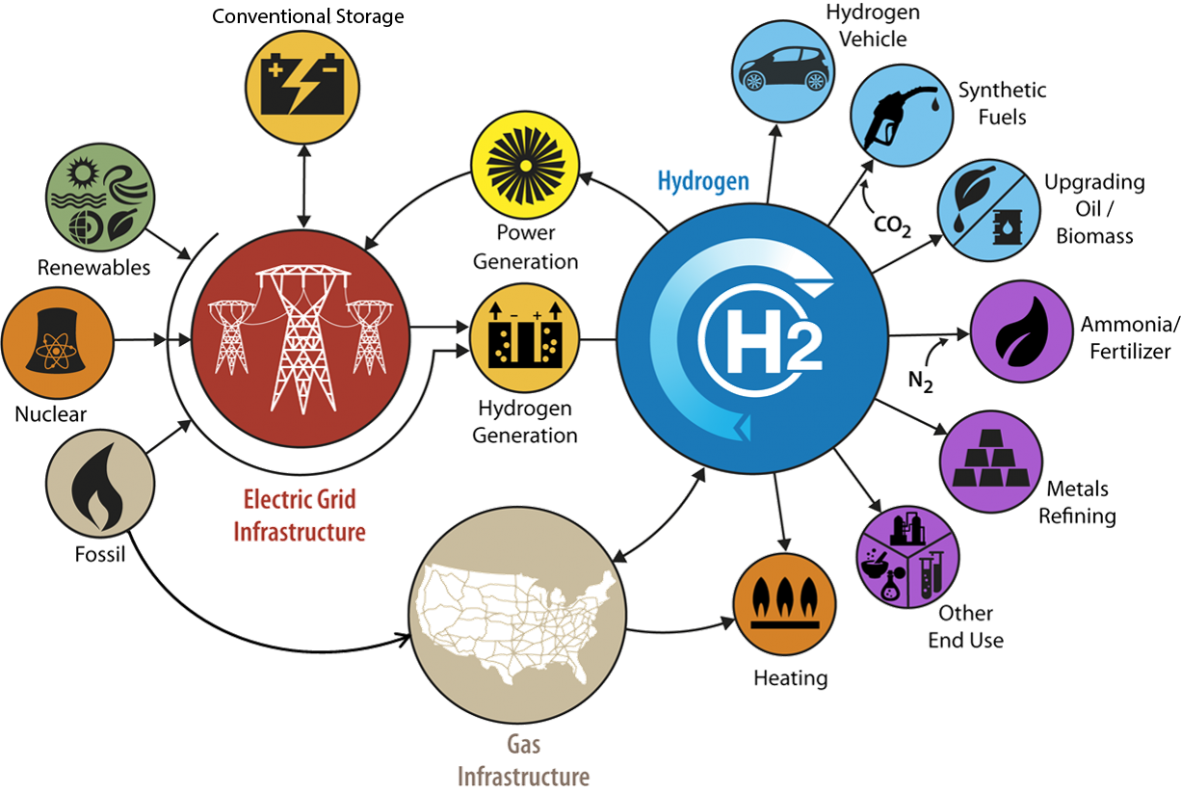
Here is a look at what hydrogen can add to the U.S. energy and fuel infrastructure. Graphic is courtesy of the Department of Energy's H2@Scale project.
Holladay is also on the steering committee for H2@Scale, where he is the lead for high-temperature hydrogen production.
H2@Scale explores the potential for manufacturing hydrogen beyond the scale that is possible now and for broadening its use in many industries.
In North America, there are just a few large-scale, energy-intensive locations that make hydrogen. About 95 percent of it comes from natural gas that is "reformed" in a chemical synthesis involving steam, a nickel catalyst, and high temperatures and pressures. From there, most of the hydrogen is used by oil-refining and ammonia industries.
That picture could be brighter. Holladay argues that hydrogen can be made from diverse, scaled-down domestic sources of energy—including wind, solar, biomass, geothermal, nuclear power, and even coal (with carbon sequestration).
By harnessing new low-cost sources of renewable energy to make hydrogen, said Holladay, “we can explore the wide-scale use of hydrogen across multiple sectors, including—and beyond—transportation.”
The H2@Scale vision includes zero-emissions vehicles, fuel cells, energy storage, steel production, food processing, and chemical production.
Low-cost hydrogen made from renewables could be put back into the energy grid, said Holladay. It could also be used to upgrade bio-oils, deployed to make cheaper ammonia, or used to make synthetic chemicals and fuels from carbon dioxide.
The Magic of Magnets
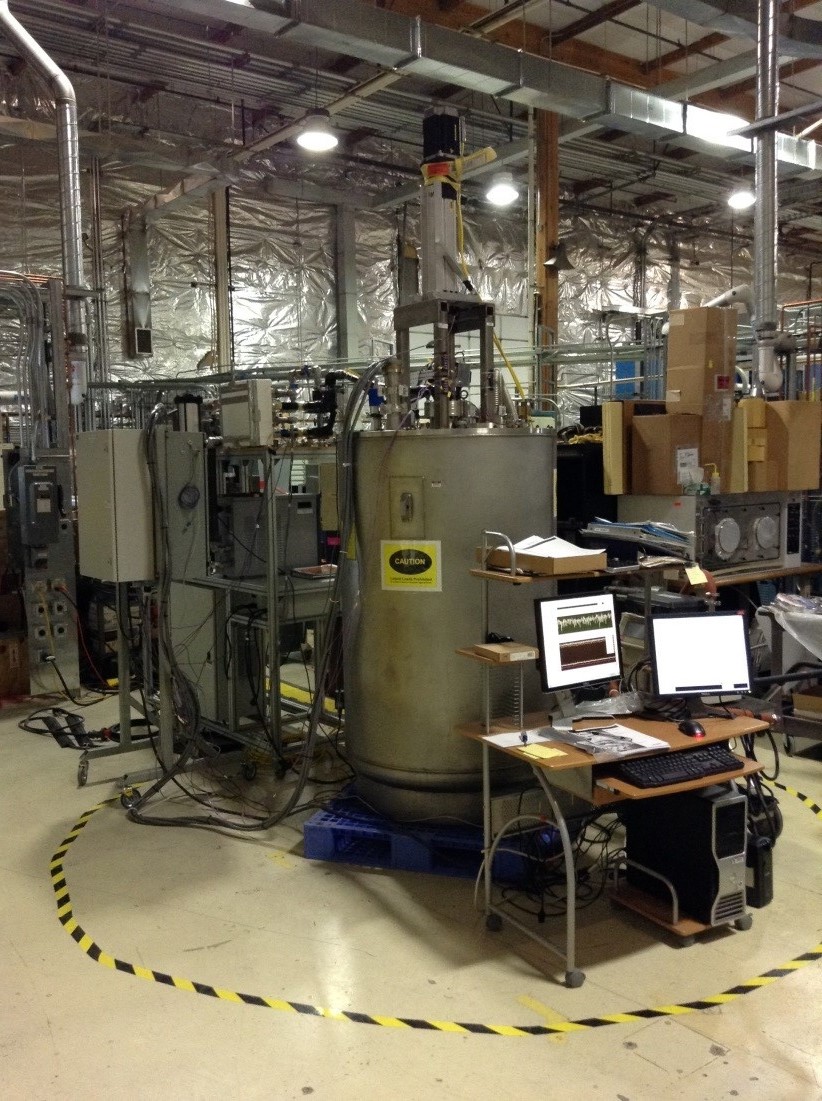
Holladay's work is central to a PNNL-designed system that uses the magnetocaloric effect to generate temperatures cold enough to liquefy gases at room temperature.
Hydrogen is the most abundant element in the universe, and its beneficial applications could include fuel cells, energy storage, petroleum recovery, food processing, and the production of methanol, ammonia, and fabricated metals. In cars and trucks, hydrogen fuel could enable zero or near-zero emissions.
However, Holladay says that making hydrogen at scale has "an Achilles heel"—the cost of cooling the gas needed to liquefy it. Current fill-up prices are close to the equivalent of $12 to $16 a gallon, which includes the cost of production, delivery, storage, compression, and dispensing.
To answer the logistics of a big part of that challenge, Holladay and his team have four projects focused on magnetic caloric hydrogen liquefaction, a technology to turn gases into liquid at room temperature by using magnets.
A piston-like fiberglass cylinder, loaded with a ferromagnetic powder like gadolinium (Gd) and gadolinium alloys, moves in an out of the magnetic field. The magnetocaloric materials heat up while in the magnetic field. When outside the field, the materials cool.
This cycle of heating and cooling can be engineered into a cooling system. With the correctly designed process, temperatures can get cold enough to liquefy gas.
Hot on Cooling’s Trail
So far, Holladay and his team have demonstrated their concept by liquefying propane to minus 55 degrees C. In recent work, they achieved minus 118 degrees C. By the end of the summer of 2019, they aspire to liquefy natural gas at approximately minus 150 degrees C.
Presently, liquefying hydrogen requires a traditional refrigeration cycle based on compressed gases. That’s energy-intensive, especially at large scales, since hydrogen must be cooled to around minus 253 degrees C (minus 423 degrees Fahrenheit).
By contrast, the magnetic-caloric technology requires less energy, is a solid-state system, and is "modular and scalable," said Holladay.
If his team can get the temperature low enough to liquefy hydrogen gas, the technology will reduce by up to 50 percent the high energy costs now required to turn hydrogen gas into an energy-bearing liquid. Projected capital cost reductions, said Holladay, may be as high as 30 percent.
Working at PNNL with Holladay on magnetic caloric hydrogen liquefaction are materials expert Kerry Meinhardt, applied chemist Evgueni Polikarpov, process engineer Kriston Brooks, and researcher Edwin Thomsen.
Partnering with Holladay and his team are the U.S. Department of Energy's Ames Laboratory in Iowa, as well as Emerald Energy NW. That company's president, John Barclay, is the inventor of active magnetic regenerators.
In all, "it’s a very exciting, dynamic time to be working in renewable energy," said Holladay. "That’s for sure."