Ending Molecular Road Rage
Wider lanes, better design could ease bumps in the road to biofuels
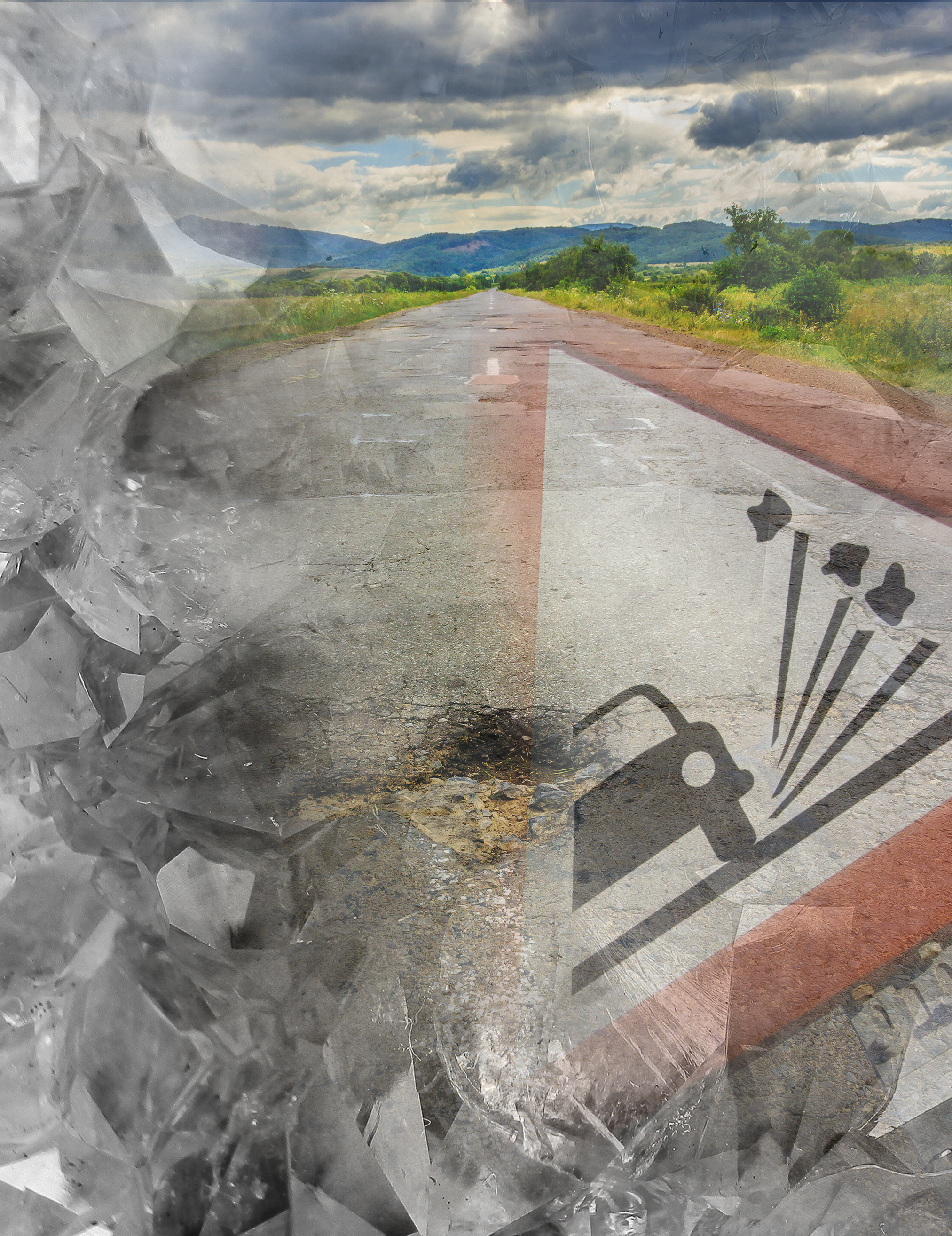
If you've ever worked in a big city, you know the highways with the too-narrow lanes or filled with potholes. These are the ones on the morning traffic report, where the highway's number is usually followed by words like "pileup," "crash," and "closed lanes." You'd avoid them if there were another route, but you need to get to work. In some ways, the same problem exists for molecules that must transit through the pores of zeolites on their path to becoming fuels. Zeolites are whitish-gray aluminosilicates, with a consistency similar to baking soda, that catalyze a host of reactions in petroleum, pharmaceutical, and possibly biofuel production.
Zeolites are regularly used because they have a host of desirable properties. These crystalline and microporous solids are formed from different aluminum and silicon sources, often in the presence of organic template. Zeolites have high thermal stability, up to 1000oC. They are used in various forms and chemical compositions. In the petroleum industry, the hydrogen form of zeolites is powerful solid state acids that catalyze cracking reactions, where long-chain hydrocarbons are broken into smaller, desirable ones. The reactions can occur at the mouth, or opening, of the zeolite's channels or deep inside. The channels in zeolites can be sized through careful synthesis, allowing only molecules of a certain size to enter and react. It is these pores and channels that are both a blessing and a curse.
"Molecules crash, stick, and generally pile up inside the zeolite channels, blocking the flow of new molecules to react and deactivate the catalyst," said Dr. Huamin Wang, a chemist who is leading zeolite studies at the Institute for Interfacial Catalysis at Pacific Northwest National Laboratory (PNNL).
The challenges continue. Zeolites work best as a powder; however, according to Heather Job, a chemist who works with zeolites in a series of high-throughput reactors at PNNL, "Powder can cause a significant drop across the reaction test bed. You are trying to flow reactants through the powder, and you have to add extra pressure."
Building Wider "Lanes" in Zeolite Catalysts. For nearly four decades, scientists at the national laboratory based in Washington State have been uncovering fundamental secrets about zeolites. They have also been answering questions for their use in emissions, petroleum, and more. For example, scientists at PNNL uncovered the fundamental reactions happening in small-pore, copper-loaded zeolites that turned toxic NOx emissions into nitrogen. The researchers improved the lifetime of the NOx catalyst, allowing it to perform at high throughputs.
For biofuel production, they are preventing the molecular pileups and making zeolites a viable catalyst for turning biological materials into fuels by creating wider channels or lanes. Researchers are starting with zeolite nanocrystals, just 20 to 100 nanometers in size.
"If we reduce the size of the starting crystal," said Dr. Miroslaw Derewinski, an expert in zeolite synthesis and characterization who recently left the Polish Academy of Sciences to join PNNL, "we will increase the number of sites that are easily accessible in the liquid phase." Working in the liquid phase rather than the gas phase means less energy and heat are needed for the reactions, increasing their overall energy efficiency.
If the channels in crystals are large, molecules entering the catalyst have less distance to travel, speeding reactions and preventing unwanted collisions. Also, the smaller crystals can suppress the extent of secondary reactions that can change the selectivity of the catalytic process.
To avoid the high pressures needed to use nanocrystals, the team used a surfactant to form a nanocrystalline solution into micelles and eventually a high-surface-area mesoporous structure. In these materials, diffusion does not play an important role in limiting the reaction. Here, the pores are an order of magnitude greater than the nanocrystals.
"We can refine mesoporous material shape," said Derewinski, "depending on the application chosen."
Not Slippery When Wet. While some forms of zeolites can withstand water, in biomass reactions water causes hydrolysis of the silicon-oxygen bond and destroys the zeolite framework. "We are combining our fundamental and applied knowledge to create more stable catalysts under reaction conditions," said Derewinski.
Researchers at PNNL are improving the stability of certain acid-based zeolites, which could effectively remove oxygen atoms from bio-oils. Created by pyrolysis of forest waste or other biological sources, bio-oils contain hydrocarbons that can become fuels. They also contain high levels of oxygenated molecules, including alcohols, ketones, and aldehydes, and a lot of water, about 40 percent by weight. The oxygen and water levels have to be reduced to create fuel. The scientists are designing stable zeolites that remove the troubling oxygen by either dehydration or decarboxylation.
"Right now, we can make water-stable zeolites, making the whole bio-oil conversion process much more efficient," said Dr. John Lee, who worked with zeolites for 30 years at Dow Chemical Company before starting on zeolite research at the national laboratory in 2009.
Traveling On. Other research at PNNL focuses on different uses of zeolites in different biofuel reactions. These include condensing oxygenates from fast pyrolysis and upgrading reactions that take short chain oxygenates, with just two or three carbons, and link them together to produce larger hydrocarbons. Other projects are improving zeolites for use in reactions that convert bio-derived syngas into aromatics and other complex hydrocarbons. "Zeolites continue to have new applications, especially in PNNL in our biofuel production research for commercial production," said Lee.
Scientists at PNNL will soon be taking on a new project that probes the chemical structure of zeolites and their behavior during reactions. These studies, including in situ examinations, are possible thanks to the high-field nuclear magnetic resonance spectroscopy, high-resolution transmission electron microscopy, and other instruments available through DOE's EMSL, a scientific user facility.
"This is simply a fascinating area of research," said Derewinski. "We've made great progress on understanding and creating better materials that are more robust and stable in water. I can't wait for what the future brings."