IIC Transformations Newletter - March 2013
Issue 2 - Feature Story
Looking at Sustainable Energy from Different Perspectives
Computational chemistry breaks bottlenecks, settles questions on intricate reactions
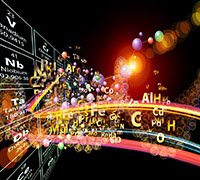
The reaction on the whiteboard of Chemistry 101 is so direct: A + B --> C + D. The reality is far more complex. Numerous intermediate steps may occur. These in-between stages can cause delays and create unwanted materials, making the overall reaction inefficient and unaffordable. Characterizing and controlling all of the steps requires more than high-resolution characterization tools.
It requires "theory."
A misnomer in some ways, theory is about applying the most fundamental principles of nature, including quantum theory, via mathematics and computer modeling to understand the changes that occur at miniscule fractions of a second at the scale of electrons, atoms and molecules. A better descriptor is "computational chemistry" or "using supercomputing power to gain insights into reactions."
At Pacific Northwest National Laboratory, computational chemistry is integrated with our laboratory experiments for sustainable energy. Our computational chemists have built models and simulations that have shown what is happening inside key reactions.
On a project with China's Dalian Institute of Chemical Physics, computational chemist Dr. Roger Rousseau and his colleagues determined why promising cobalt catalysts failed to efficiently turn biomass-derived synthesis gas into alcohols needed as fuel additives.
"The modeling was very instructive. It was fascinating to look at why we were getting different results," said Dr. Jonathan Male, an experimentalist who worked on the project and now leads PNNL's biomass client
On another project, PNNL scientists conducted experiments using rhodium-based catalysts to convert synthesis gas into oxygen-rich chemicals for fuels. The catalyst did poorly. Iterating between computational chemistry and characterization tools, the team generated hypotheses about how the catalyst's three main components interacted with each other and with the synthesis gas. By understanding the catalyst's tendencies, the team made significant improvements. The result? A long-lasting catalyst and much higher yields.
"Without the theory, we would have had to do this almost entirely by trial and error," said Mark Gerber, the project manager. "We wouldn't have known what was happening at the molecular level or why."
Computational chemistry is also important in PNNL's studies into basic scientific principles. Dr. Simone Raugei, Dr. Wendy Shaw and their colleagues are learning how proton delivery and removal influences the speed of a nickel-based catalyst. The catalyst drives a reaction that produces or breaks hydrogen, a vital reaction in fuel cells and biofuels. "To get catalysts to achieve their full potential, we need to understand all of the bottlenecks and how to overcome them," said Shaw.
"When we started on the research, there was the belief that breaking or forming hydrogen was the crucial step," said Raugei. "It isn't. It is putting protons in the right spot on the catalyst. Once you have them in the right spot, everything goes very quickly."
And the same can be said of scientists; once you have computational chemists and laboratory experimentalists in the right spot – together – everything goes very quickly.