Magnetic Resonance Imaging of Porous Membranes
(February 2006)
Nuclear magnetic resonance (NMR) is a spectroscopic technique for probing the chemical state of certain nuclei, such as hydrogen, that have a magnetic moment. The nuclei may be naturally occurring, such as water in a biological specimen, or may be added to a specimen to outline structures of interest. When a material containing these nuclei is placed in a magnetic field and subjected to electromagnetic radiation, a resonance condition will occur at a particular frequency, causing the spin state of the nucleus to flip. The resonant frequency is determined by the local chemical environment in which the nucleus resides. A spectrum results with a sharp absorption line at the resonance frequency. Magnetic resonance imaging (MRI) processes multiple NMR scans of a specimen and uses computer processing to construct a series of images of thin slices of the specimen. Subtle variations in the local chemical environment are readily detected by this non-invasive method that allows acquisition of successive 3-D images at a nominal resolution of tens of micrometers in a matter of seconds.
MRI is a valuable tool for the characterization of buried interfaces in complex materials and devices. For example, evaluation of pore size and connectivity has been demonstrated in polymer membranes used in filtering and sequestration applications and as ionic electrolytes in fuel cells. A grand challenge for improving the performance of polymer membrane fuel cells involves understanding gas flow dynamics, reactive site homogeneity, and the formation mechanism and transport of water through the ion-conducting membrane during operation. Magnetic resonance spectroscopy now enables such investigations to be performed on operating cells as a means to understand gas flow properties and the ubiquitous role of water on fuel cell performance and materials reliability.
The usefulness of hyperpolarized 129Xe NMR spectroscopy to characterize molecular adsorption in ultraporous polymers and molecular diffusion dynamics through the interconnected pore space has been demonstrated for resorcinol-formaldehyde aerogels. (Fig 1.) Gradual heating of cooled aerogel samples into which both adsorbant (CHCl3) and Xe probe atoms have been infused, actuates diffusion within the pore space accompanied by changes in the measured xenon chemical shift and site residence time from which information on pore size, size distribution, and pore connectivity can be extracted.
Based on the 129Xe and related work, hyperpolarized 3He NMR measurements were used to image gas flow through PEM fuel cells in real time to provide the experimental information necessary to validate computational fluid dynamics simulations (Fig. 2, Fig. 3). The high sensitivity of proton NMR to selectively detect water, and –OH offers a 3-D imaging tool to understand water transport phenomena and chemical reactivity at the membrane electrode interface.
The non-invasive nature, ready availability of robust instrumentation, and the high information content of acquired data, make MRI an attractive and complementary alternative to neutron scattering and/or highly instrumented fuel cells for correlating interfacial reactivity and attendant ion and molecular transport phenomena with fuel cell performance. Real-time images of both gas flow and water distribution in the cell are critical to both understanding and managing electrolyte transport characteristics with major impact on fuel cell design and associated efficiency.
This research was conducted by Li-Qiong Wang, Kevin Minard, Peter Rieke, Paul Major, Vilayanur Viswanathan, Gregory J. Exarhos, and Richard Jacob, Pacific Northwest National Laboratory; Theodore F. Baumann and Joe Satcher, Lawrence Livermore National Laboratory; and Igor L. Moudrakovski, Christopher I. Ratcliffe, and John A. Ripmeester, NRC, Canada.
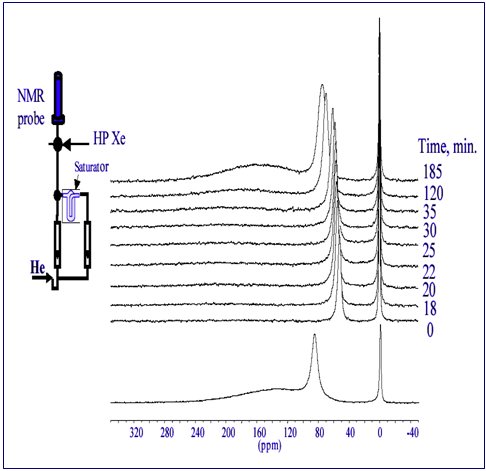
Figure 1. Adsorption of guest molecules (CHCl3) in nanopore cavities is evaluated by 129Xe NMR measurements using xenon tracer atoms. (A gas mixture is introduced to samples in a closed system by means of the apparatus shown in the inset.) Xe chemical shift and line intensities are used to determine analyte pore filling characteristics. Upon cessation of analyte flow, desorption occurs as a function of time and voided pores become available for Xe residency. The magnitude of the chemical shift correlates with pore size.
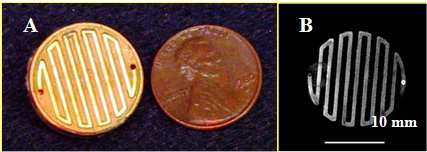
Figure 2. A) Gold-coated gas manifold for a micro PEM fuel cell in comparison with a penny. B) Representative magnetic resonance image of 3He gas mixed with 10% nitrogen flowing through the same manifold at atmospheric pressure.
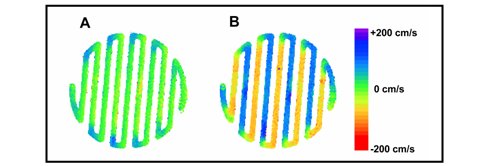
Figure 3. Color-coded maps of 3He gas flow velocity measured in the A) horizontal and B) vertical directions. Image data in B shows how the flow velocity changes direction as the gas traverses the serpentine path of the manifold during normal operation.