Motivating Carbon Dioxide
Scientists show what it takes to get the potential fuel feedstock to a reactive spot on a model catalyst
(April 2013)
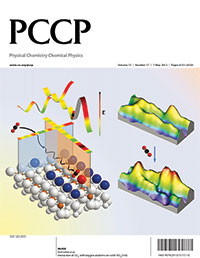
The research graced the cover of Physical Chemistry Chemical Physics. Image reproduced by permission of Zdenek Dohnalek and The Royal Society of Chemistry from Physical Chemistry Chemical Physics 15:6190-6195. DOI: 10.1039/c3cp44040k Enlarge Image
Results: In extreme cold, carbon dioxide huddles near charged oxygen atom outcroppings on the surface of oft-studied titanium dioxide; the carbon dioxide lacks the energy to reach a more protected spot, according to scientists at Pacific Northwest National Laboratory. When heated, the carbon dioxide slides into a more substantial, reactive oxygen vacancy, holes left by missing oxygen atoms. The team tracked the carbon dioxide with a scanning tunneling microscope tip that provided atomic-resolution images.
"Scientists often focus on oxygen vacancies, because they are very active," said Dr. Xiao Lin, a Linus Pauling Distinguished Postdoctoral Fellow at PNNL, who spent the last year working on this study. "We focused on the oxygen adatom with atomic resolution and got rather surprising results."
Why It Matters: Replacing gasoline with fuels built using carbon dioxide and solar energy could greatly increase our nation's energy independence. This process requires new processes and photocatalysts, long-lasting materials that use light to drive the reaction. Determining the barriers to getting carbon dioxide into the oxygen vacancies, where the photocatalyzed reactions often occur, is a fundamental step in creating the needed materials for solar fuels.
"Carbon dioxide and titanium dioxide is not the ideal marriage in terms of anything more applied," said Dr. Zdenek Dohnalek, an experimental chemist on the study. "But, because titanium dioxide is so fundamental, it gives generally applicable insights at a very basic level."
Methods: In their studies the researchers devised a new approach that allowed them to change the contrast of oxygen atoms on the surface. To achieve this atomic-resolution view they modified a standard scanning tunneling microscope tip by attaching a single carbon dioxide molecule at the end.
"It actually makes a big difference in how the oxygen atoms appear in the image," said Dohnalek.
Because the surface flaws and other characteristics of titanium dioxide are well documented, the team used this material. The titanium dioxide surface consists of rows of oxygen atoms stacked on top of titanium atoms. Two common defects on otherwise perfect structure of the surface: adatoms and vacancies. Adatoms are stray, negatively charged oxygen atoms that rest on the surface. Vacancies are gaps in the oxygen rows, created when an oxygen atom is missing.
Working at 50 K, or around -369°F, the researchers added the carbon dioxide to the surface. They found that the carbon dioxide did not have the energy to move into the oxygen vacancies, instead resting near the adatoms.
"If you absorb carbon dioxide at a low temperature, there are kinetic limitations - energy hills -- that the molecules can't get over to get into the vacancies," said Lin.
Surprisingly, the team found that the carbon dioxide molecules near the adatoms were influenced by nearby oxygen vacancies and carbon dioxide molecules. With the new imaging approach, they showed how the molecule near the adatom swung in a crescent-shaped path. If an oxygen vacancy existed one to four atoms away, the molecule tilted away from it. If another carbon dioxide molecule was directly across from it, the carbon dioxide molecule would tilt towards it.
"These are long-range effects on the tiniest of scales," said Dohnalek.
When the scientists subsequently warmed the surface to 100 to 160 K, around the temperature of a night on the moon, the carbon dioxide moved into the vacancies. The molecules overcame the kinetic energy barrier.
"The discoveries needed to solve today's problems aren't something that you're going to get by eyeballing a sample and analyzing a spreadsheet," said Dr. Louis Terminello, who leads the Chemical Imaging Initiative at PNNL. "You need new approaches and new ideas."
What's Next? Having proven the value of the new chemical imaging approach and determined how carbon dioxide behaved on a model oxide semiconductor at low temperatures, the team is now applying the technique to ruthenium dioxide. Metallic ruthenium dioxide, an electricity conductor, is structurally similar to titanium dioxide, but very different chemically. Adding to the understanding of these materials gives scientists the foundation necessary for the coming catalytic breakthroughs necessary to turn sunlight into fuels.
Acknowledgments:
Sponsors: Linus Pauling Distinguished Postdoctoral Fellowship Program funded by Laboratory Directed Research and Development (LDRD) Program at PNNL (Xiao Lin); Chemical Imaging Initiative funded by the LDRD Program at PNNL (Zhi-Tao Wang, Igor Lyubinetsky); the U.S. Department of Energy, Office of Basic Energy Sciences, Chemical Sciences, Geosciences & Biosciences Division (Bruce D. Kay, Zdenek Dohnalek)
Research Area: Chemical Imaging and Chemical Sciences
User Facility: Environmental Molecular Sciences Laboratory
Research Team: Xiao Lin, Zhi-Tao Wang, Igor Lyubinetsky, Bruce D. Kay, and Zdenek Dohnalek, PNNL
Reference: Lin X, ZT Wang, I Lyubinetsky, BD Kay, and Z Dohnalek. 2013. "Interaction of CO2 with Oxygen Adatoms on Rutile TiO2(110)." Physical Chemistry Chemical Physics 15:6190-6195. DOI: 10.1039/c3cp44040k
Related Highlight: The Complexities of a Simple Molecule